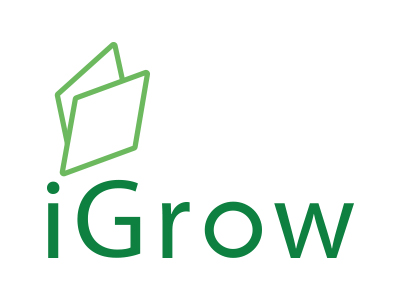
Welcome to iGrow News, Your Source for the World of Indoor Vertical Farming
Signify and RIAT Pioneer Growing Tomatoes and Cucumbers In A Vertical Farm Without Daylight
Signify (Euronext: LIGHT), the world leader in lighting, and RIAT, an innovative farming enterprise located in Russia, have together pioneered the growing of tomatoes and cucumbers in a vertical farm without daylight.
April 30, 2020
Eindhoven, the Netherlands – Signify (Euronext: LIGHT), the world leader in lighting, and RIAT, an innovative farming enterprise located in Russia, have together pioneered the growing of tomatoes and cucumbers in a vertical farm without daylight. During the research phase in 2019, RIAT achieved a yield comparable to advanced traditional greenhouses using Philips GreenPower LED grow lights.
We already had facilities of sufficient height at our disposal, therefore there was no need to invest in building a glass construction. But as the building had no windows, we needed a high-quality lighting system to fulfill the intensive lighting requirements of tomatoes and cucumbers. In 2019, Signify provided its Philips GreenPower LED grow lighting, helping us to achieve a yield performance comparable to traditional greenhouse operations."
Vladimir Bukharev
Director at RIAT
RIAT’s indoor farm is located in the center of the Ivanovo region, enabling the company to distribute its tomatoes, cucumbers and 19 types of green crops to its own RIAT stores within an hour after harvesting. RIAT has a special technique to fold the long stems, allowing it to grow the 3.5m high plants indoors.
“With a harvest of 2.7 to 3.2 kg of cucumbers per m2 every week, and 1.7 kg per m2 of tomatoes, we started making a profit as of the second year of operation,” Bukharev added. “In addition to that, the quality of the product is very high, there’s hardly any waste and we sell 99% of what is being grown.”
“To mimic nature as much as possible, RIAT has chosen a combination of different kinds of Philips GreenPower LED toplighting and interlighting, bringing light both from the top and right in the canopy where the light is needed the most for photosynthesis. While using different kinds of lights, the light recipe can be adjusted based on the crop that is grown,” said Sergey Khokhrin, business Development Manager CEE/Russia & CIS at Signify.
Signify, in collaboration with several research partners, discovered that the red spectrum in light stimulates growth of plant cells and tissues, while the blue spectrum influences the processes of cytodifferentiation (racemes initiation, bines and root formation, flowering). The combination of red and blue with the addition of white and other colors in Philips LED grow lights creates lighting conditions that are as close to natural daylight as possible. Additionally, RIAT is using bumblebees for pollination, as in traditional greenhouses. The bees don’t experience difficulties due to the absence of natural light.
When choosing a lighting supplier, RIAT tested LED modules from different manufacturers from around the globe. “By using Philips LED lighting modules, we managed to achieve maximum stability and a light output of 18 g/mol. This is why we chose Signify. The area equipped with LED lamps is currently 3,800 m2. Soon, we are going to launch a new area of 700 m2 to grow lettuce which will also be equipped with Philips LED lighting,” Bukharev added.
For further information, please contact:
Global Marcom Manager Horticulture at Signify
Daniela Damoiseaux
Tel: +31 6 31 65 29 69
E-mail: daniela.damoiseaux@signify.com
Signify Corporate Communications and Government Affairs in Russia
and CIS
Natalia Neverskaya
Tel.: +7 (495) 937-93-30; fax +7 (495) 937-93-59
E-mail: natalia.neverskaya@signify.com
About Signify
Signify (Euronext: LIGHT) is the world leader in lighting for professionals and consumers and lighting for the Internet of Things. Our Philips products, Interact connected lighting systems and data-enabled services, deliver business value and transform life in homes, buildings and public spaces. With 2019 sales of EUR 6.2 billion, we have approximately 38,000 employees and are present in over 70 countries. We unlock the extraordinary potential of light for brighter lives and a better world. We have been named Industry Leader in the Dow Jones Sustainability Index for three years in a row. News from Signify is located at the Newsroom, Twitter, LinkedIn and Instagram. Information for investors can be found on the Investor Relations page.
Micro-Naps For Plants: Flicking The Lights On And Off Can Save Energy Without Hurting Indoor Agriculture Harvests
A nighttime arrival at Amsterdam’s Schiphol Airport flies you over the bright pink glow of vegetable production greenhouses. Growing crops under artificial light is gaining momentum, particularly in regions where produce prices can be high during seasons when sunlight is sparse.
The Netherlands is just one country that has rapidly adopted controlled-environment agriculture, where high-value specialty crops like herbs, fancy lettuces and tomatoes are produced in year-round illuminated greenhouses. Advocates suggest these completely enclosed buildings – or plant factories – could be a way to repurpose urban space, decrease food miles and provide local produce to city dwellers.
One of the central problems of this process is the high monetary cost of providing artificial light, usually via a combination of red and blue light-emitting diodes. Energy costs sometimes exceed 25% of the operational outlay. How can growers, particularly in the developing world, compete when the sun is free? Higher energy use also translates to more carbon emissions, rather than the decreased carbon footprint sustainably farmed plants can provide.
I’ve studied how light affects plant growth and development for over 30 years. I recently found myself wondering: Rather than growing plants under a repeating cycle of one day of light and one night of darkness, what if the same daylight was split into pulses lasting only hours, minutes or seconds?
Short bursts of light and dark
So my colleagues and I designed an experiment. We’d apply the normal amount of light in total, just break it up over different chunks of time.
Of course plants depend on light for photosynthesis, the process that in nature uses the sun’s energy to merge carbon dioxide and water into sugars that fuel plant metabolism. Light also directs growth and development through its signals about day and night, and monkeying with that information stream might have disastrous results.
That’s because breaking something good into smaller bits sometimes creates new problems. Imagine how happy you’d be to receive a US$100 bill – but not as thrilled with the equivalent 10,000 pennies. We suspected a plant’s internal clock wouldn’t accept the same luminous currency when broken into smaller denominations.
And that’s exactly what we demonstrated in our experiments. Kale, turnip or beet seedlings exposed to cycles of 12 hours of light, 12 hours dark for four days grew normally, accumulating pigments and growing larger. When we decreased the frequency of light-dark cycles to 6 hours, 3 hours, 1 hour or 30 minutes, the plants revolted. We delivered the same amount of light, just applied in different-sized chunks, and the seedlings did not appreciate the treatment.
The same amount of light applied in shorter intervals over the day caused plants to grow more like they were in darkness. We suspect the light pulses conflicted with a plant’s internal clock, and the seedlings had no idea what time of day it was. Stems stretched taller in an attempt to find more light, and processes like pigment production were put on hold.
But when we applied light in much, much shorter bursts, something remarkable happened. Plants grown under five-second on/off cycles appeared to be almost identical to those grown under the normal light/dark period. It’s almost like the internal clock can’t get started properly when sunrise comes every five seconds, so the plants don’t seem to mind a day that is a few seconds long.
Just as we prepared to publish, undergraduate collaborator Paul Kusuma found that our discovery was not so novel. We soon realized we’d actually rediscovered something already known for 88 years. Scientists at the U.S. Department of Agriculture saw this same phenomenon in 1931 when they grew plants under light pulses of various durations. Their work in mature plants matches what we observed in seedlings with remarkable similarity.
A 1931 study by Garner and Allard tracked the growth of Yellow Cosmos flowers under light pulses of various durations. J. Agri. Res. 42: National Agricultural Library, Agricultural Research Service, U.S. Department of Agriculture., CC BY-ND
Not only was all of this a retread of an old idea, but pulses of light do not save any energy. Five seconds on and off uses the same amount of energy as the lights being on for 12 hours; the lights are still on for half the day.
But what would happen if we extended the dark period? Five seconds on. Six seconds off. Or 10 seconds off. Or 20 seconds off. Maybe 80 seconds off? They didn’t try that in 1931.
Building in extra downtime
It turns out that the plants don’t mind a little downtime. After applying light for five seconds to activate photosynthesis and biological processes like pigment accumulation, we turned the light off for 10, or sometimes 20 seconds. Under these extended dark periods, the seedlings grew just as well as they had when the light and dark periods were equal. If this could be done on the scale of an indoor farm, it might translate to a significant energy savings, at least 30% and maybe more.
Recent yet-to-be published work in our lab has shown that the same concept works in leaf lettuces; they also don’t mind an extended dark time between pulses. In some cases, the lettuces are green instead of purple and have larger leaves. That means a grower can produce a diversity of products, and with higher marketable product weight, by turning the lights off.
One variety of lettuce grew purple when given a 10-second dark period. They look similar to those grown with a five-second dark period, yet use 33% less energy. Extending the dark period to 20 seconds yielded green plants with more biomass. J. Feng, K. Folta
Learning that plants can be grown under bursts of light rather than continuous illumination provides a way to potentially trim the expensive energy budget of indoor agriculture. More fresh vegetables could be grown with less energy, making the process more sustainable. My colleagues and I think this innovation could ultimately help drive new business and feed more people – and do so with less environmental impact.
July 22, 2019 6.58am EDT Updated July 22, 2019 2.40pm EDT
This article was updated with a corrected legend on the photograph of the plants grown in 1931.
CO2 Enrichment, Acclimation, And Efficiency In Hydroponic Growing
Carbon dioxide (CO2) is an essential requirement for photosynthesis and can be somewhat overlooked by newer growers. Being odorless, invisible, and only a small fraction of our atmosphere, CO2 often doesn’t get the same attention as nutrients, lights, and other plant-growth factors
Lynette Morgan | August 23, 2019
Takeaway: While many commercial growers use carbon dioxide to boost crop quality, yield, and growth rates, Lynette Morgan explains how home hydroponic horticulturists can also take advantage of CO2 enrichment.
Carbon dioxide (CO2) is an essential requirement for photosynthesis and can be somewhat overlooked by newer growers. Being odorless, invisible, and only a small fraction of our atmosphere, CO2 often doesn’t get the same attention as nutrients, lights, and other plant-growth factors.
The use of CO2 enrichment to boost yields, quality, and growth rates under hydroponic production is, however, widely used in commercial greenhouse horticulture and has an even greater potential in enclosed growing spaces. While simply pumping in some additional CO2 may seem like a straightforward option, the use of this technology is a little more complex if its potential is to be maximized and problems minimized.
CO2 Enrichment
Ambient CO2 levels in air are a little more than 400 ppm (or 0.04 per cent by volume), however, plant tissue contains an average of 45 per cent carbon that comes entirely from CO2. By boosting CO2 levels surrounding the leaf surface, above ambient levels, the rate of photosynthesis increases up until the point where some other factor, such as the speed at which plant enzymes will work, is reached.
Email Newsletter
Join thousands of other growers who are already receiving our monthly newsletter.
Essentially, the transfer of CO2 from the surrounding air to the reaction centers in the leaf chloroplasts depends both on the concentration difference between the air and these sites, and the intervening biochemical resistance in various leaf tissues. This means that while CO2 enrichment will boost photosynthesis, there comes a point were further increases will not occur and plant damage becomes a possibility. Determining this optimal level of CO2 enrichment for a particular plant or stage of growth is where the application of CO2 needs some careful thought.
Carbon dioxide enrichment has become more popular in recent times with hydroponic growers using a range of low- and high-tech options to boost CO2 levels. The most common methods of generating CO2 include burning hydrocarbon fuels and the use of compressed, bottled CO2. Smaller growers with a very limited growing space may use dry ice (solid, very cold CO2) which releases CO2 as it “melts” under warm conditions.
Fermentation or the decomposition of organic matter (composting and fungi) are still effective but less accurate ways of boosting CO2 levels through natural processes. Whichever method is used to generate CO2, levels should be regularly monitored, either with a hand-held CO2 meter or as part of the environmental control system in the growing area.
Read also: The Benefits of Adding CO2 During the Cloning Stage
Enrichment levels
If CO2 enrichment is to be applied, then determining the correct level is as important with this gaseous element as it is with nutrient levels. The benefits and levels of CO2enrichment is crop dependent, but most plants respond well to levels in the range of 500–1,500ppm. Below 200ppm, CO2 begins to severely limit plant growth, but more than 2,000ppm of CO2 becomes toxic to many plants.
More than 4,000ppm is a risk to humans. An excess of CO2 will cause crop damage in the form of CO2 toxicity, which is often misdiagnosed as mineral deficiencies or disease symptoms. Mild CO2 toxicity can cause stunting of growth, or leaf-aging type symptoms, while excessive levels may cause leaf damage such as chlorosis (yellowing), necrosis (death of leaf tissue), curling and/or thickening of the leaves.
There is much debate over which level of enrichment is ideal for each crop, under various different growing conditions, however, the most economic use of CO2 is in enriching crops to above ambient levels, but not more than 1,200ppm. Most commercial growers enrich to within the range of 600-800ppm where an increase in growth and yields of between 20-30 per cent are common.
While CO2 enrichment is largely used on fruiting crops such as tomatoes, capsicum, and cucumber, it can benefit a wide range of plant species. Indoor gardens with ornamental, potted, and flowering plants also respond to CO2 enrichment with increased rates of growth and leaf area, increased rates of flowering, more lateral breaks, earlier flowering, greater flower number, reduced flower drop, and increased flower diameter as well as improved leaf color and reduced time to maturity. Carbon dioxide also assists with root development on cuttings and clones in many species and may be applied via enrichment of the air or through the use of carbonated mist.
CO2 Efficiency
To make the most of CO2 enrichment, other growth factors need to be considered and manipulated. Carbon dioxide enrichment will produce the best results in terms of plant growth, yield increases, and shortening the time to maturity where there is high light to power rapid levels of photosynthesis.
If light is insufficient or below the light saturation point for the crop, then boosted CO2levels cannot be fully utilized by the plants. Temperature also plays a role in the efficient use of CO2. Under conditions of high light and CO2 enrichment, temperatures can be run higher than they would normally, and this maximizes the effect of additional CO2.
Studies have shown that for tomato plants, a threefold level of CO2 enrichment will increase net photosynthesis by about 50 per cent in both dull and bright light, but if leaf temperature is also raised (to 86°F), the increase in net CO2 fixation can be as high as 100 per cent in bright light. This means that while boosting CO2 in an indoor hydroponic system will boost growth rates, consideration should be given at the same time to manipulation of the other environmental factors of light and temperature if the valuable CO2 is to be used with the highest degree of efficiency.
Another often overlooked factor is CO2 distribution around the plants. Simply releasing or generating CO2 for enrichment into the growing area is often not sufficient to get the maximum rate of photosynthesis unless this is directed and circulated over leaf surfaces. A stale boundary layer of moist air, depleted in CO2 due to photosynthesis, can form directly around the leaf surface and this needs frequent removal and replenishment.
Whatever source of CO2 generation is being used, it is vital that the enriched atmosphere is thoroughly mixed so the valuable CO2 is delivered to plant surfaces for uptake and assimilation. Small mixer fans can be used to gently circulate the air away from the source of CO2 generation and toward the crop.
To monitor this process, hand-held CO2 meters are useful to check levels in and around the canopy rather than just at the point of CO2 release. Keeping a check on CO2 levels inside a small growing area is vitally important, no matter what the source of CO2 used. It can be difficult to judge how much CO2 the plants are taking up and in tightly sealed growing environments, CO2 accumulation can occur and cause plant damage.
Read also: The Symbiotic Relationship Between CO2 and Ventilation
CO2 Acclimation
CO2 enrichment is undoubtedly a great growth-promoting tool for hydroponic growers, however, it has its limitations and risks.
Plants have the ability to adjust and adapt to increasing CO2 levels, so that over time, acclimation occurs. When CO2 enrichment is first introduced to a crop, there is a rapid increase in photosynthesis and growth, but as plant growth continues, the effect of the increased CO2 levels becomes less and less so that by the time the crop is completed, overall yields were not as high as the increase in early yield.
Numerous studies have reported this effect with plants grown continuously at high CO2 levels having a photosynthetic rate that tends to decrease with time. If a crop grown at elevated CO2 levels is suddenly given only ambient CO2, it will recover back to normal rates of photosynthesis within five days.
Some growers have attempted to prevent this acclimation of crops to high CO2 levels by only supplying CO2 intermittently, or avoiding the use of CO2 enrichment until a vital stage of development, such as flowering or fruit set, has been reached when the boost in photoassimilate is most valuable to yields. Studies have shown the problem of CO2 acclimation can be reduced or eliminated if the plant has strong “sinks” for the assimilate produced in the leaves.
These sinks for assimilate include rapidly developing tissues such as buds, flowers, and fruits. Plants with a low sink strength often end up with carbohydrate accumulating in the leaves under CO2 enrichment, which in turn triggers acclimation and a reduction in photosynthesis. Despite the issue of plant acclimation to high CO2levels limiting the overall potential boost to growth, CO2-enriched plants still produce photosynthetic rates higher than those grown at ambient CO2 levels.
Carbon dioxide enrichment is a worthwhile tool for indoor and greenhouse growers which is well proven in a wide range of crop species to increase growth rates and yields. However, as with most high-tech techniques, it requires monitoring, attention to detail, and careful consideration of the effect on biochemical processes. If CO2 is to be used at maximum efficiency, correct rates of application, adjustments to light and temperature, timing of enrichment, and consequences of CO2 acclimation all need consideration.
Written by Lynette Morgan
Dr. Lynette Morgan holds a B. Hort. Tech. degree and a PhD in hydroponic greenhouse production from Massey University, New Zealand. Lynette is a partner with Suntec International Hydroponic Consultants and has authored several hydroponic technical books. Visit suntec.co.nz for more information. Full Bio
Micro-Naps For Plants: Flicking The Lights On And Off Can Save Energy Without Hurting Indoor Agriculture Harvests
Growing crops under artificial light is gaining momentum , particularly in regions where produce prices can be high during seasons when sunlight is sparse
7/22/2019
Author: Kevin M. Folta
(MENAFN - The Conversation) A nighttime arrival at Amsterdam's Schiphol Airport flies you over the bright pink glow of vegetable production greenhouses. Growing crops under artificial light is gaining momentum , particularly in regions where produce prices can be high during seasons when sunlight is sparse.
The Netherlands is just one country that has rapidly adopted controlled-environment agriculture , where high-value specialty crops like herbs, fancy lettuces and tomatoes are produced in year-round illuminated greenhouses.Advocates suggest these completely enclosed buildings – or plant factories– could be a way to repurpose urban space, decrease food miles and provide local produce to city dwellers.
One of the central problems of this process is the high monetary cost of providing artificial light , usually via a combination of red and blue light-emitting diodes.Energy costs sometimes exceed 25% of the operational outlay. How can growers, particularly in the developing world,compete when the sun is free ? Higher energy use also translates to more carbon emissions, rather than the decreased carbon footprint sustainably farmed plants can provide.
I'vestudied how light affects plant growth and development for over 30 years. I recently found myself wondering: Rather than growing plants under a repeating cycle of one day of light and one night of darkness, what if the same daylight was split into pulses lasting only hours, minutes or seconds?
Indoor plants need plenty of artificial light. josefkubes/Shutterstock.com
Short bursts of light and dark
So my colleagues and I designed an experiment . We'd apply the normal amount of light in total, just break it up over different chunks of time.
Of course plants depend on light for photosynthesis, the process that in nature uses the sun's energy to merge carbon dioxide and water into sugars that fuel plant metabolism. Light also directs growth and development through its signals about day and night, and monkeying with that information stream might have disastrous results.
That's because breaking something good into smaller bits sometimes creates new problems. Imagine how happy you'd be to receive a US$100 bill – but not as thrilled with the equivalent 10,000 pennies. We suspected a plant's internal clock wouldn't accept the same luminous currency when broken into smaller denominations.
And that's exactly what we demonstrated in our experiments . Kale, turnip or beet seedlings exposed to cycles of 12 hours of light, 12 hours dark for four days grew normally, accumulating pigments and growing larger. When we decreased the frequency of light-dark cycles to 6 hours, 3 hours, 1 hour or 30 minutes, the plants revolted. We delivered the same amount of light, just applied in different-sized chunks, and the seedlings did not appreciate the treatment.
The same amount of light applied in shorter intervals over the day caused plants to grow more like they were in darkness. We suspect the light pulses conflicted with aplant's internal clock , and the seedlings had no idea what time of day it was. Stems stretched taller in an attempt to find more light, and processes like pigment production were put on hold.
But when we applied light in much, much shorter bursts, something remarkable happened. Plants grown under five-second on/off cycles appeared to be almost identical to those grown under the normal light/dark period. It's almost like the internal clock can't get started properly when sunrise comes every five seconds, so the plants don't seem to mind a day that is a few seconds long.
Just as we prepared to publish, undergraduate collaborator Paul Kusuma found that our discovery was not so novel. We soon realized we'd actually rediscovered something already known for 88 years. Scientists at the U.S. Department of Agriculture saw this same phenomenon in 1931 when they grew plants under light pulses of various durations. Their work in mature plants matches what we observed in seedlings with remarkable similarity.
A 1931 study by Garner and Allard tracked the growth of Yellow Cosmos flowers under light pulses of various durations.
J. Agri. Res. 42: National Agricultural Library, Agricultural Research Service, U.S. Department of Agriculture.,CC BY
Not only was all of this a retread of an old idea, but pulses of light do not save any energy. Five seconds on and off uses the same amount of energy as the lights being on for 12 hours; the lights are still on for half the day.
But what would happen if we extended the dark period? Five seconds on. Six seconds off. Or 10 seconds off. Or 20 seconds off. Maybe 80 seconds off? They didn't try that in 1931.
Building in extra downtime
It turns out that the plants don't mind a little downtime. After applying light for five seconds to activate photosynthesis and biological processes like pigment accumulation, we turned the light off for 10, or sometimes 20 seconds. Under these extended dark periods, the seedlings grew just as well as they had when the light and dark periods were equal. If this could be done on the scale of an indoor farm, it might translate to a significant energy savings, at least 30% and maybe more.
Recent yet-to-be published work in our lab has shown that the same concept works in leaf lettuces; they also don't mind an extended dark time between pulses. In some cases, the lettuces are green instead of purple and have larger leaves. That means a grower can produce a diversity of products, and with higher marketable product weight, by turning the lights off.
One variety of lettuce grew purple when given a 10-second dark period. They look similar to those grown with a five-second dark period, yet use 33% less energy. Extending the dark period to 20 seconds yielded green plants with more biomass.
J. Feng, K. Folta
Learning that plants can be grown under bursts of light rather than continuous illumination provides a way to potentially trim the expensive energy budget of indoor agriculture. More fresh vegetables could be grown with less energy, making the process more sustainable. My colleagues and I think this innovation could ultimately help drive new business and feed more people – and do so with less environmental impact.
Hacking Photosynthesis
Artificial photosynthesis: can we harness the energy of the sun as well as plants?
13 January, 2019
We will all be dead without the Sun. That we all know. But even if the sun shone 24 hours a day, we will all be dead without plants. Really. Plants keep the world going. We eat a lot of plants – and the animals from which we obtain meat for consumption also consume plants. Furthermore, plants inhale Carbon Dioxide and produce healthier air. The process through which plants get the energy for sustenance (and all other stuff) is called Photosynthesis which means something like ‘producing with light’.
This is fundamental to the life cycle on Earth. But how does photosynthesis work? There’s a big molecule, a protein, inside the leaves of most plants. It is called Rubisco. It is probably the most abundant protein in the world. Rubisco has one job.
It picks up carbon dioxide from the air, and it uses the carbon to make sugar molecules. It gets the energy to do this from the Sun. This is photosynthesis, the process by which plants use sunlight to make food, the foundation of life on Earth.
But Rubisco is not perfect. It has one almost fatal flaw. Unfortunately, Rubisco does not know how to grab only Carbon Dioxide from the air. It also picks up oxygen. But this poses a huge problem for the plant as this leads to the formation of a toxic compound in the plant. It has to do extra work and spend extra energy for detoxification, a process called Photorespiration. This has in fact been called ‘one of the biggest mistakes’ of evolution.
Ripe
Plants have a complicated ‘chemical assembly line’ in their cells to carry out this detoxification, but the process uses up a lot of energy. This means the plant has less energy for actually making food. However, some crops including corn and sugar cane have developed a workaround for Rubisco, making them much more productive. Photorespiration is anti-photosynthesis in the sense that it costs the plant precious energy and resources that it could have invested in photosynthesis to produce more growth and yield.
Many scientists around the world have been trying to ‘hack’ photosynthesis for years, but a team from the University of Illinois has emerged first. There, a research program called Realizing Increased Photosynthetic Efficiency (RIPE), has run for the last five years trying to fix Rubisco’s problem.
They first experimented with tobacco plants, because tobacco is easy to work with. The researchers inserted some new genes into these plants, which shut down the existing detoxification assembly line and set up a new one that is much more efficient.
Photorespiration normally takes a complicated route through three compartments in the plant cell. Scientists engineered alternate pathways to reroute the process, drastically shortening the trip and saving enough resources to boost plant growth.
This resulted in super tobacco plants that grow faster and up to 40 percent bigger than normal tobacco plants. And yes, this was not confined to the laboratory.
These measurements were done both in greenhouses and open-air farm plots. Their research has been published in the prestigious Science magazine.
These scientists now are trying to repeat the process with plants that people rely on for food, such as, tomatoes and soybeans.
They will also be working with cowpea, or black-eyed pea, which is a staple food crop for a lot of farmers in sub-Saharan Africa. One can indeed imagine the effects of more efficient photosynthesis in the poorest regions of the world. The funders of this project include the U.S. Department of Agriculture and the Bill and Melinda Gates Foundation.
It will be many years, though, before any farmer anywhere in the world plant crops with this new version of photosynthesis. Researchers will have to find out whether it means that a food crop actually produces a bigger harvest, while convincing Government regulators and consumers that the crops are safe to grow and eat.
There is an irrational fear regarding Genetically Modified Organism (GMO) food, even though there is no conclusive evidence that they cause disease or deformities. The public however should be assured that the plants with the photosynthesis hack pose no danger to people and animals.
Precision Agriculture
In any case, photosynthesis is just one component of a plant’s needs. Plant growth, in man-made fields or in the wild, depends on the availability of water, nitrogen and phosphorus, not on photosynthetic capacity alone. Farmers generally add water and NPK fertiliser to their crops, though wild plants have to find these on their own. And lest someone think that the sun is essential for photosynthesis for all plants, plenty of plants grow well under artificial light. In fact, indoor agriculture has been proposed as one solution to the impending food crisis in some parts of the world. Moreover, soil-less and artificial light plant growth will be essential for future manned space missions being planned for Mars.
Agriculture is ripe for modernisation in many developing parts of the world where crop yields are still low compared to those of the developed world. Boosting crop yields is essential with the world predicted to have 10 billion people by 2050.
That is three billion extra mouths to feed and a possible 70 percent extra demand for food, but arable land is not getting any bigger. The solution is to improve crop yields and also adopt innovative methods of agriculture such as vertical farming, soil-less farming and indoor farming.
And there is a whole new revolution coming to traditional agriculture too – including self-driving tractors and harvesters, crop-spraying drones, robots, Artificial Intelligence and satellite sensing. There is even a name for agriculture that combines the best elements of technology - precision agriculture.
Internet of Things
The goal is to use automated driving technology, computer vision, telematics, and cloud-based mobile applications to help farmers double or triple their yields—a feat that will be key to keeping up with global food demands as the Earth’s population grows over the next 30 years.
The Internet of Things (IOT) will also help agriculture. Some machines are stuffed with sensors and software that gather data, process it with machine learning, and beam it into mobile apps. The sensors are the eyes of the machine. The software and mobile apps bring the data to life.
The other major challenge is Climate Change, which has the potential to cause a severe disruption to our crop cycles. A Recent analysis that looked into the climate impact on crop yields produced sobering results.
The study’s authors said that for each 1° Celsius rise in global mean temperature there would be a 7.4% decrease in yields of corn, a 6% decrease in yields of wheat, and a 3% yield decrease in rice. It is thus vital to keep Climate Change in check as other advances could be nullified if it reaches unmanageable levels.
Video: Scientists Soup Up Photosynthesis
Oceanographer Penny Chisholm introduces us to an amazing little being: Prochlorococcus, the most abundant photosynthetic species on the planet. A marine microbe that has existed for millions of years, Prochlorococcus wasn't discovered until the mid-1980s -- but its ancient genetic code may hold clues to how we can reduce our dependence on fossil fuels.
Penny Chisholm · Microbial oceanographer, author
Penny Chisholm studies an extremely tiny microorganism that plays an enormous role in ocean ecosystems. Discovered only three decades ago, it has defined her career and inspired her to think differently about life on Earth.