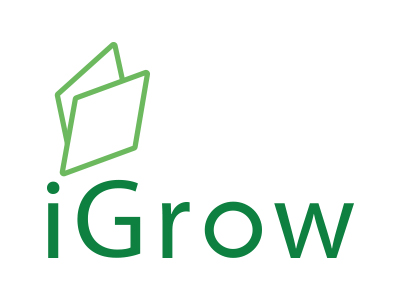
Welcome to iGrow News, Your Source for the World of Indoor Vertical Farming
MIT Engineers Have Discovered A Completely New Way of Generating Electricity
A new material made from carbon nanotubes can generate electricity by scavenging energy from its environment
By ANNE TRAFTON
MASSACHUSETTS INSTITUTE OF TECHNOLOGY
JUNE 7, 2021
MIT engineers have discovered a way to generate electricity using tiny carbon particles that can create an electric current simply by interacting with an organic solvent in which they’re floating. The particles are made from crushed carbon nanotubes (blue) coated with a Teflon-like polymer (green). Credit: Jose-Luis Olivares, MIT. Based on a figure courtesy of the researchers.
Tiny Particles Power Chemical Reactions
A new material made from carbon nanotubes can generate electricity by scavenging energy from its environment.
MIT engineers have discovered a new way of generating electricity using tiny carbon particles that can create a current simply by interacting with liquid surrounding them.
The liquid, an organic solvent, draws electrons out of the particles, generating a current that could be used to drive chemical reactions or to power micro- or nanoscale robots, the researchers say.
“This mechanism is new, and this way of generating energy is completely new,” says Michael Strano, the Carbon P. Dubbs Professor of Chemical Engineering at MIT. “This technology is intriguing because all you have to do is flow a solvent through a bed of these particles. This allows you to do electrochemistry, but with no wires.”
In a new study describing this phenomenon, the researchers showed that they could use this electric current to drive a reaction known as alcohol oxidation — an organic chemical reaction that is important in the chemical industry.
Strano is the senior author of the paper, which appears today (June 7, 2021) in Nature Communications. The lead authors of the study are MIT graduate student Albert Tianxiang Liu and former MIT researcher Yuichiro Kunai. Other authors include former graduate student Anton Cottrill, postdocs Amir Kaplan and Hyunah Kim, graduate student Ge Zhang, and recent MIT graduates Rafid Mollah and Yannick Eatmon.
Unique properties
The new discovery grew out of Strano’s research on carbon nanotubes — hollow tubes made of a lattice of carbon atoms, which have unique electrical properties. In 2010, Strano demonstrated, for the first time, that carbon nanotubes can generate “thermopower waves.” When a carbon nanotube is coated with layer of fuel, moving pulses of heat, or thermopower waves, travel along the tube, creating an electrical current.
That work led Strano and his students to uncover a related feature of carbon nanotubes. They found that when part of a nanotube is coated with a Teflon-like polymer, it creates an asymmetry that makes it possible for electrons to flow from the coated to the uncoated part of the tube, generating an electrical current. Those electrons can be drawn out by submerging the particles in a solvent that is hungry for electrons.
To harness this special capability, the researchers created electricity-generating particles by grinding up carbon nanotubes and forming them into a sheet of paper-like material. One side of each sheet was coated with a Teflon-like polymer, and the researchers then cut out small particles, which can be any shape or size. For this study, they made particles that were 250 microns by 250 microns.
When these particles are submerged in an organic solvent such as acetonitrile, the solvent adheres to the uncoated surface of the particles and begins pulling electrons out of them.
“The solvent takes electrons away, and the system tries to equilibrate by moving electrons,” Strano says. “There’s no sophisticated battery chemistry inside. It’s just a particle and you put it into solvent and it starts generating an electric field.”
“This research cleverly shows how to extract the ubiquitous (and often unnoticed) electric energy stored in an electronic material for on-site electrochemical synthesis,” says Jun Yao, an assistant professor of electrical and computer engineering at the University of Massachusetts at Amherst, who was not involved in the study. “The beauty is that it points to a generic methodology that can be readily expanded to the use of different materials and applications in different synthetic systems.”
Particle power
The current version of the particles can generate about 0.7 volts of electricity per particle. In this study, the researchers also showed that they can form arrays of hundreds of particles in a small test tube. This “packed bed” reactor generates enough energy to power a chemical reaction called an alcohol oxidation, in which alcohol is converted to an aldehyde or a ketone. Usually, this reaction is not performed using electrochemistry because it would require too much external current.
“Because the packed bed reactor is compact, it has more flexibility in terms of applications than a large electrochemical reactor,” Zhang says. “The particles can be made very small, and they don’t require any external wires in order to drive the electrochemical reaction.”
In future work, Strano hopes to use this kind of energy generation to build polymers using only carbon dioxide as a starting material. In a related project, he has already created polymers that can regenerate themselves using carbon dioxide as a building material, in a process powered by solar energy. This work is inspired by carbon fixation, the set of chemical reactions that plants use to build sugars from carbon dioxide, using energy from the sun.
In the longer term, this approach could also be used to power micro- or nanoscale robots. Strano’s lab has already begun building robots at that scale, which could one day be used as diagnostic or environmental sensors. The idea of being able to scavenge energy from the environment to power these kinds of robots is appealing, he says.
“It means you don’t have to put the energy storage onboard,” he says. “What we like about this mechanism is that you can take the energy, at least in part, from the environment.”
Reference: “Solvent-induced electrochemistry at an electrically asymmetric carbon Janus particle” by Albert Tianxiang Liu, Yuichiro Kunai, Anton L. Cottrill, Amir Kaplan, Ge Zhang, Hyunah Kim, Rafid S. Mollah, Yannick L. Eatmon, and Michael S. Strano, 7 June 2021, Nature Communications.
DOI: 10.1038/s41467-021-23038-7
The research was funded by the U.S. Department of Energy and a seed grant from the MIT Energy Initiative.
A Monster Wind Turbine Is Upending An Industry
Twirling above a strip of land at the mouth of Rotterdam’s harbor is a wind turbine so large it is difficult to photograph. The turning diameter of its rotor is longer than two American football fields end to end
G.E.’s Giant Machine, Which
Can Light Up A Small Town,
Is Stoking A Renewable-Energy Arms Race
By Stanley Reed
January 1, 2021
Twirling above a strip of land at the mouth of Rotterdam’s harbor is a wind turbine so large it is difficult to photograph. The turning diameter of its rotor is longer than two American football fields end to end. Later models will be taller than any building on the mainland of Western Europe.
Packed with sensors gathering data on wind speeds, electricity output and stresses on its components, the giant whirling machine in the Netherlands is a test model for a new series of giant offshore wind turbines planned by General Electric. When assembled in arrays, the wind machines have the potential to power cities, supplanting the emissions-spewing coal- or natural gas-fired plants that form the backbones of many electric systems today.
G.E. has yet to install one of these machines in ocean water. As a relative newcomer to the offshore wind business, the company faces questions about how quickly and efficiently it can scale up production to build and install hundreds of the turbines.
But already the giant turbines have turned heads in the industry. A top executive at the world’s leading wind farm developer called it a “bit of a leapfrog over the latest technology.” And an analyst said the machine’s size and advance sales had “shaken the industry.”
A New Generation of Wind Turbines
General Electric’s prototype for a new offshore wind turbine, the Haliade-X, is the largest ever built.
London Eye - Ferris wheel GE Haliade-X 13MW - wind turbine Empire State Building
HEIGHT: 443 feet HEIGHT: 853 feet HEIGHT: 1,050 feet
Source: General Electric
The prototype is the first of a generation of new machines that are about a third more powerful than the largest already in commercial service. As such, it is changing the business calculations of wind equipment makers, developers, and investors.
The turning diameter of the Haliade-X’s rotor is longer than two American football fields.Credit...Ilvy Njiokiktjien for The New York Times
The G.E. machines will have a generating capacity that would have been almost unimaginable a decade ago. A single one will be able to turn out 13 megawatts of power, enough to light up a town of roughly 12,000 homes.
The turbine, which is capable of producing as much thrust as the four engines of a Boeing 747 jet, according to G.E., will be deployed at sea, where developers have learned that they can plant larger and more numerous turbines than on land to capture breezes that are stronger and more reliable.
The race to build bigger turbines has moved faster than many industry figures foresaw. G.E.’s Haliade-X generates almost 30 times more electricity than the first offshore machines installed off Denmark in 1991.
In coming years, customers are likely to demand even bigger machines, industry executives say. On the other hand, they predict that, just as commercial airliners peaked with the Airbus A380, turbines will reach a point where greater size no longer makes economic sense.
“We will also reach a plateau; we just don’t know where it is yet,” said Morten Pilgaard Rasmussen, chief technology officer of the offshore wind unit of Siemens Gamesa Renewable Energy, the leading maker of offshore turbines.
The turbine is so large that it will be able to generate enough power to light up a town of 12,000 homes. Credit...Ilvy Njiokiktjien for The New York Times
Although offshore turbines now account for only about 5 percent of the generating capacity of the overall wind industry, this part of the business has taken on an identity of its own and is expected to grow faster in the coming years than land-based wind.
Offshore technology took hold in Northern Europe in the last three decades and is now spreading to the East Coast of the United States as well as Asia, including Taiwan, China and South Korea. The big-ticket projects costing billions of dollars that are possible at sea are attracting large investors, including oil companies like BP and Royal Dutch Shell, that want to quickly enhance their green energy offerings. Capital investment in offshore wind has more than tripled over the last decade to $26 billion, according to the International Energy Agency, the Paris-based forecasting group.
G.E. began making inroads in wind power in 2002 when it bought Enron’s land-based turbine business — a successful unit in a company brought down in a spectacular accounting scandal — at a bankruptcy auction. It was a marginal force in the offshore industry when its executives decided to try to crack it about four years ago. They saw a growing market with only a couple of serious Western competitors.
Still, G.E.’s bosses figured that to become a leader in the more challenging marine environment, they needed to be audacious. They proceeded to more than double the size of their existing offshore machine, which came to G.E. through its acquisition of the power business of France’s Alstom in 2015. The idea was to gain a lead on key competitors like Siemens Gamesa and Vestas Wind Systems, the Danish-based turbine maker.
A larger turbine produces more electricity and, thus, more revenue than a smaller machine. Size also helps reduce the costs of building and maintaining a wind farm because fewer turbines are required to produce a given amount of power.
These qualities create a powerful incentive for developers to go for the largest machine available to aid their efforts to win the auctions for offshore power supply deals that many countries have adopted. These auctions vary in format, but developers compete to provide power over a number of years for the lowest price.
The prototype is on a piece of land in Rotterdam Harbor, but the Haliade-X is designed as an offshore turbine, to be planted in relatively shallow sea water. Credit...Ilvy Njiokiktjien for The New York Times
“What they are looking for is a turbine that allows them to win these auctions,” said Vincent Schellings, who has headed design and production of the G.E. turbine. “That is where turbine size plays a very important role.”
Among the early customers is Orsted, a Danish company that is the world’s largest developer of offshore wind farms. It has a preliminary agreement to buy about 90 of the Haliade-X machines for a project called Ocean Wind off Atlantic City, N.J.
“I think they surprised everybody when they came out with that machine,” said David Hardy, chief executive of Orsted’s offshore business in North America.
As a huge buyer of turbines, Orsted wants to help “establish this new platform and create some volume for G.E.” so as to promote competition and innovation, Mr. Hardy said.
The G.E. turbine is selling better than its competitors may have expected, analysts say.
On Dec. 1, G.E. reached another preliminary agreement to provide turbines for Vineyard Wind, a large wind farm off Massachusetts, and it has deals to supply 276 turbines to what is likely to be the world’s largest wind farm at Dogger Bank off Britain.
One day turbines will reach a size limit, but “we just don’t know where it is yet,” said Morten Pilgaard Rasmussen of Siemens Gamesa Renewable Energy. Credit...Carsten Snejbjerg for The New York Times
These deals, with accompanying maintenance contracts, could add up to $13 billion, estimates Shashi Barla, principal wind analyst at Wood Mackenzie, a market research firm.
The waves made by the G.E. machine have pushed Siemens Gamesa to announce a series of competing turbines. Vestas, which until recently had the industry’s biggest machine in its stable, is also expected to unveil a new entry soon.
“We didn’t move as the first one, and that of course we have to address today,” said Henrik Andersen, the chief executive of Vestas.
To pull off its gambit, G.E. had to start “pretty much from scratch,” Mr. Schellings said. The business unit called G.E. Renewable Energy is spending about $400 million on design, hiring engineers and retooling factories at St. Nazaire and Cherbourg in France.
To make a blade of such extraordinary length that doesn’t buckle from its own weight, G.E. called on designers at LM Wind Power, a blade maker in Denmark that the company bought in 2016 for $1.7 billion. Among their innovations: a material combining carbon fiber and glass fiber that is lightweight yet strong and flexible.
Offshore turbines account for only about 5 percent of the generating capacity of the overall wind industry, but that number is expected to grow. Credit...Ilvy Njiokiktjien for The New York Times
G.E. still must work out how to manufacture large numbers of the machines efficiently, initially at the plants in France and, possibly later, in Britain and the United States. With a skimpy offshore track record, G.E. also needs to show that it can reliably install and maintain the big machines at sea, using specialized ships and dealing with rough weather.
“G.E. has to prove a lot to asset owners for them to procure G.E. turbines,” Mr. Barla said.
Bringing out bigger machines has been easier and cheaper for Siemens Gamesa, G.E.’s key rival, which is already building a prototype for a new and more powerful machine at its offshore complex at Brande on Denmark’s Jutland peninsula. The secret: The company’s ever-larger new models have not strayed far from a decade-old template.
“The fundamentals of the machine and how it works remain the same,” said Mr. Rasmussen, the unit’s chief technology officer, leading to a “starting point that was a little better” than G.E.’s.
There seems to be plenty of room for competition. John Lavelle, the chief executive of G.E.’s offshore business, said the outlook for the market “gets bigger each year.”
Stanley Reed has been writing from London for The Times since 2012 on energy, the environment and the Middle East. Prior to that, he was London bureau chief for BusinessWeek magazine. @stanleyreed12 • Facebook
A version of this article appears in print on Jan. 2, 2021, Section B, Page 1 of the New York edition with the headline: A Monster Wind Turbine Is Upending an Industry. Order Reprints | Today’s Paper | Subscribe
New Partnership Brings Clean Energy To Indoor Farming
The benefits of indoor farming–including chemical-free food production unrestricted by seasonality, climate change and water scarcity–have been recognized globally and are driving rapid industry growth
Hydroponic vertical container farming company Freight Farms and Arcadia, a monthly subscription service connecting renters and homeowners across the U.S. to clean energy, have partnered to provide Freight Farms’ U.S. customers with access to clean energy for their everyday operations. With this partnership, Freight Farms and Arcadia are taking the first critical stride to align their respective industries, moving indoor farming into a more sustainable future.
The benefits of indoor farming–including chemical-free food production unrestricted by seasonality, climate change and water scarcity–have been recognized globally and are driving rapid industry growth. While it has made significant advancements in resource efficiency, the industry continues to struggle with the sustainability of electrical power use.
Together, these two companies are moving towards addressing this limitation by connecting Freight Farmers to affordable clean power at a time when the nation’s grid is dominated by fossil fuel. With an Arcadia membership, Freight Farmers can choose to match their electrical use with wind and solar energy, which will also help create more demand for clean energy providers overall.
Without changing anything in their day-to-day operations. Freight Farms’ customers can now connect the utility for their container farm to Arcadia to match 100% of the farm’s electrical usage with clean energy.
Clean energy accessibility
“Our farmers are passionate about sustainability by nature of their efforts to grow healthy food hyper-locally. But many are unable to adopt clean energy directly based on cost and availability of options in their location,” said Rick Vanzura, Freight Farms’ CEO. “With Arcadia, our farmers are able to further reduce their business’ carbon footprint while simultaneously increasing demand for more clean energy in the market.”
“Arcadia was built so that anyone anywhere can use our platform to access clean energy,” added Alexa Minerva, senior director of partnerships at Arcadia. “We’re excited about partnering with Freight Farms to make it possible for farmers to reap the benefits of renewables, potentially save money and combat the effects of climate change.”
Freight Farms’ modular container model makes this partnership uniquely possible within the indoor farming industry, as large agricultural enterprises use too much energy for community solar projects, which are capped at a relatively small size by state law.
Connecting to clean energy
Without changing anything in their day-to-day farm operations, Freight Farms’ customers can now connect their utility through Arcadia in two minutes. Upon connection, Arcadia will begin matching 100 percent of the Freight Farm’s electricity by purchasing an equivalent amount of wind and solar energy in the form of Renewable Energy Certificates (RECs). The result reduces Freight Farmers’ carbon footprint to one-quarter of industrial farming operations. Based on location and other factors, Arcadia can also help farmers save on their electricity bills.
Memberships are available in two options:
As enabled by state law, farmers located in MA, RI, NY, IL, CO, MD and ME can sign up to access the community solar power market and will see a reduction in their electricity bills.
All other U.S. Freight Farmers can sign up for $5/month to access clean energy.
Innovation in sustainability
The partnership with Arcadia is the latest initiative in Freight Farms’ history. Freight Farms’ Greenery has been involved with technological advances driving greater sustainability within the sector. The Greenery uses 98.9 percent less water than industrial farming--even achieving water-positive operations in certain locations. The Greenery’s proprietary fixed lighting arrays also leverage LED market technology to triple light energy output without an increased corresponding energy draw. The result is a growing platform that pairs the highest potential yields with resource efficiency.
Freight Farms’ pioneering modular design enables hyper-local farming anywhere, including harsh climates and urban areas lacking land access, reducing food production carbon impact in other ways as well. Transportation missions are reduced or eliminated and irrigation isn’t necessary. Hyper-local farming also reduces food waste by providing consumers just-picked produce with freshness, flavor and shelf life, says the company.
15 Dec 2020
Engineers Found A Way to Generate Electricity From Thin Air
If it can be scaled up, Air-gen technology could power everything from iPhones to car charging stations
Green energy, as far as we’ve implemented it, is at best a shade of greenish-brown — army, perhaps, or olive. Solar farms harness the sun’s renewable energy but require large swaths of land and rare Earth metals. Wind power has a minimal carbon footprint, but, like solar, gets stored in batteries made from lead and lithium. Nuclear power is appealingly low carbon, but the risk of another Chernobyl is hard to stomach. All of these options are a huge improvement over coal power, but there’s real pressure to find an energy source that’s truly scalable, cheap, and 100% green.
Last week, scientists from the University of Massachusetts Amherst rose to the challenge, presenting a low-cost device they call the Air-gen, which generates electricity from thin air — enough to theoretically power devices like cellphones and electric cars. And since it doesn’t require harsh chemicals to produce, “the whole process,” corresponding author and assistant electrical engineer professor Jun Yao tells OneZero via email, is “green.”
Improbable as it sounds, the device’s technology is based on a natural phenomena: electricity-generating threads of proteins, called nanowires, that emanate out of a tiny bacteria called Geobacter sulfurreducens (a plush toy version of the bacteria looks like a Cheeto with tentacles). In the paper they published in the journal Nature last week, Yao and his co-authors describe their key discovery: Moisture suspended naturally in the air is the “driving force” behind the electricity-generating ability of the nanowires.
Think back to high school physics. Electricity is basically the flow of electrons, the negatively charged particles that circle atoms, from an area of high charge to an area of low charge. Water can be a good source of these charged electrons if it can be broken up into its building blocks, hydrogen, and oxygen.
The team theorizes that when a tangle of the nanowires is pressed into a mesh-like film, water from the air collects only at the top of it and breaks up into two Hs and an O, freeing up the water’s electrons. This gives the top of the film a greater charge than the bottom, setting up the perfect conditions for electrons to flow. When the researchers sandwiched one of these films between two gold electrodes to create a circuit, the tiny device produced a voltage of 0.5 volts across a film just seven micrometers thick. (A charged car battery, for comparison, measures at about 12.6 volts.)
“The whole process is green.”
Essentially, the Air-gen can generate a flow of electrons without much input, thanks to the impressive biology of Geobacter’s nanowires. Co-author and distinguished microbiology professor Derek Lovely, who has dedicated more than 30 years of study to the electric microbe, compares the wires to individual human hairs — a thread made up of different proteins, only 20,000 times thinner than what you’d pluck out of your scalp.
Before we can slap patches of these nanowire films onto an iPhone or Fitbit, their production will need to be scaled up so that multiple patches can work in tandem to generate enough electricity to power modern devices. Figuring out how to mass-produce the nanowires, plus devising a “clever engineering strategy” to put them together in a compact way, says Yao, will be key.
They’ve already started tackling the former problem by attempting to cut Geobacter bacteria itself out of the picture. “It is not easy to make large quantities of wires with Geobacter,” explains Lovley. Some bacteria are easier to grow en masse than others. Because it grows so quickly, E. coli has become scientists’ go-to for genetic engineering. Lovley’s team engineered a strain of E. coli that produces Geobacter’s nanowires, so now they have a ready supply.
“Now that we have solved the microbiological bottleneck, the engineers can begin designing larger Air-gen devices,” he says.
There’s still the question of whether the technology is as miraculously green as it seems to be. Yao maintains that production of the nanowires is completely harmless to the environment — and cheap to boot — because “we simply feed renewable feedstocks into the bacteria without harsh/toxic chemical involved.” The cost, according to an estimate from Lovley, would be 100-fold lower than that needed to make semiconductor-grade silicon, which is used for solar cells.
From the nanowires to the way they’re produced to the energy generated, says Yao, “All are green.” When the green label is placed on technology, he points out, it often “only means that the energy production part is green, and can still generate e-waste.”
Lovley says that there isn’t any existing technology that resembles Air-gen, as far as he’s aware. There doesn’t appear to be a patent on the Air-gen at the time of this article’s publication, but the research was funded by a seed fund through UMass-Amherst’s Office of Technology Commercialization and Ventures. The team appears set to scale up quickly.
The way Yao sees it, powering a large device with the Air-gen is only a matter of adding up many tiny generators. It’s analogous to using “thousands of battery cells” to “drive a Tesla car,” even though each individual battery cell only has limited energy. They can potentially power small-scale wearables, medium-scale electric tools, or even a remote station, as long as they can scale quickly. And they seem confident that they can.
In a cheeky tweet last week, he sent a “final response” to reviewers of their Nature paper: a gif of Gene Wilder in Young Frankenstein, mouthing his iconic line “It could work!”
“Now that we can make more wires, addressing this question should be relatively straight forward,” Lovley says.
OneZero
The undercurrents of the future. A Medium publication about tech and science.
Engineering Electricity Energy Science And Medicine Power
Lead Image: UMass Amherst/Yao and Lovley Labs
WRITTEN BY
Yasmin Tayag
Senior editor at OneZero at Medium. Previously at Inverse. Covering all science that’s shaping the future.
OneZero
The undercurrents of the future. A Medium publication about tech and science.
Device Recycles Waste Heat Into Light To Boost Solar Systems
Arrays of aligned single-wall carbon nanotubes could channel wasted heat and greatly raise the efficiency of solar energy systems, report researchers
JULY 16TH, 2019
Arrays of aligned single-wall carbon nanotubes could channel wasted heat and greatly raise the efficiency of solar energy systems, report researchers.
The new invention is a hyperbolic thermal emitter that can absorb intense heat that would otherwise spew into the atmosphere, squeeze it into a narrow bandwidth, and emit it as light that can be turned into electricity.
The discovery rests on another that Junichiro Kono’s group at the Brown School of Engineering at Rice University made in 2016 when it found a simple method to make highly aligned, wafer-scale films of closely packed nanotubes.
A scanning electron microscope image shows submicron-scale cavities patterned into films of aligned carbon nanotubes. The cavities trap thermal photons and narrow their bandwidth, turning them into light that can then be recycled as electricity. (Credit: Naik Lab)
WASTE HEAT
Discussions with Gururaj Naik, an assistant professor of electrical and computer engineering, led the pair to see if the films could be used to direct “thermal photons.”
“Thermal photons are just photons emitted from a hot body,” Kono says. “If you look at something hot with an infrared camera, you see it glow. The camera is capturing these thermally excited photons.”
ABOUT 20 PERCENT OF OUR INDUSTRIAL ENERGY CONSUMPTION IS WASTE HEAT. THAT’S ABOUT THREE YEARS OF ELECTRICITY JUST FOR THE STATE OF TEXAS.
Infrared radiation is a component of sunlight that delivers heat to the planet, but it’s only a small part of the electromagnetic spectrum.
“Any hot surface emits light as thermal radiation,” Naik says. “The problem is that thermal radiation is broadband, while the conversion of light to electricity is efficient only if the emission is in a narrow band. The challenge was to squeeze broadband photons into a narrow band.”
The nanotube films presented an opportunity to isolate mid-infrared photons that would otherwise be wasted. “That’s the motivation,” Naik says. “A study by [co-lead author and graduate student] Chloe Doiron found that about 20 percent of our industrial energy consumption is waste heat. That’s about three years of electricity just for the state of Texas. That’s a lot of energy being wasted.
CARBON NANOTUBES CAN TAKE THE HEAT
“The most efficient way to turn heat into electricity now is to use turbines, and steam or some other liquid to drive them,” he says. “They can give you nearly 50 percent conversion efficiency. Nothing else gets us close to that, but those systems are not easy to implement.” Naik and his colleagues aim to simplify the task with a compact system that has no moving parts.
The aligned nanotube films are conduits that absorb waste heat and turn it into narrow-bandwidth photons. Because electrons in nanotubes can only travel in one direction, the aligned films are metallic in that direction while insulating in the perpendicular direction, an effect Naik called hyperbolic dispersion. Thermal photons can strike the film from any direction, but can only leave via one.
“Instead of going from heat directly to electricity, we go from heat to light to electricity,” Naik says. “It seems like two stages would be more efficient than three, but here, that’s not the case.”
Naik says adding the emitters to standard solar cells could boost their efficiency from the current peak of about 22 percent. “By squeezing all the wasted thermal energy into a small spectral region, we can turn it into electricity very efficiently,” he says. “The theoretical prediction is that we can get 80 percent efficiency.”
Nanotube films suit the task because they stand up to temperatures as high as 1,700 degrees Celsius (3,092 degrees Fahrenheit). Naik’s team built proof-of-concept devices that allowed them to operate at up to 700 C (1,292 F) and confirm their narrow-band output. To make them, the team patterned arrays of submicron-scale cavities into the chip-sized films.
“There’s an array of such resonators, and each one of them emits thermal photons in just this narrow spectral window,” Naik says. “We aim to collect them using a photovoltaic cell and convert it to energy, and show that we can do it with high efficiency.”
A paper on the technology appears in ACS Photonics. The Basic Energy Science program of the Department of Energy, the National Science Foundation, and the Robert A. Welch Foundation supported the research.
Source: Rice University
Lead Photo: (Credit: James Moran/Flickr)
Original Study DOI: acsphotonics.9b00452
TAGS
History of Microgrids In The US: From Pearl Street To Plug-and-Play
While it may seem that microgrids are new, the history of microgrids shows they have been around in some form for years in the US
July 22, 2019
By Lisa Cohn
While it may seem that microgrids are new, the history of microgrids shows they have been around in some form for years in the US — although they haven’t always been called microgrids. The first one was introduced by Thomas Edison in 1882 at his Pearl Street Station, which combined heat and power and produced electricity and thermal energy.
The Battery and Control Room in the first Edison Electric Lighting Station at Pearl Street in lower Manhattan in 1882. By Everett Historical/Shutterstock.com
In fact, campuses have been using microgrids for decades and have shown that microgrids are compatible with local utility grids and provide benefits to both the campuses and the larger grids.
Universities were ideal early adapters of microgrids because they have large, easily defined loads. In addition, many campuses have physical plants that provide steam for heating.
As a result, an upgrade to combined heat and power (CHP) microgrid makes sense in many cases. CHP technology allows them to produce both electricity and steam from a single fuel, which dramatically boosts the efficiency of the system.
Campuses with microgrids include Wesleyan University, Harvard University, Princeton University and the University of California at San Diego (UCSD). One of the biggest microgrids in the US is at the University of Texas at Austin, which can supply all of the university’s power, heating and cooling needs.
In the late 1990s, Congress was concerned about the reliability of national electricity transmission, and asked the U.S. Department of Energy (DOE) for guidance. The conversation focused on maximizing distributed generation to reduce stress on the grid. A number of research projects were launched over the years, leading to demonstration projects of microgrid technology for utilities, universities, industry, school districts, jails, hospitals, laboratories, military bases and industrial parks.
Pivotal event in history of microgrids: Superstorm Sandy
A series of severe storms from 2011-2012 in the Northeast heightened interest in microgrids, the most destructive being Superstorm Sandy. Microgrid operators, like Princeton University, showcased how microgrid technology kept power on when the central grid failed during Sandy.
Efforts to rebuild the electricity infrastructure prompted people to ask questions about how to better prepare in the future. This helped raise awareness about microgrids and distributed energy.
A handful of states played a big role in the history of microgrids, among them California, Connecticut, illinois, Massachuetts, New Jersey and New York.
For example, in 2013 Connecticut became the first state to offer microgrid funding when it announced its Microgrid Pilot Program. Nine microgrid projects were awarded $18 million in funding through the first round.
The program is now in its fourth round of funding, awarding up to $30 million to microgrid projects. Award recipients have included two campuses, Trinity College and Wesleyan University, a family-owned dairy farm and pet shelter, the town of Coventry, and even an apartment complex.
The Ameren Microgrid in Champaign, Illinois, August 2017. Photo courtesy Ameren Illinois
In 2014, New York created the New York Prize, a $40 million competition launched to offer money to those who plan on developing community microgrids. The initiative was created to find microgrids that could be easily replicated and used as models for other communities nationwide.
On the other side of the country, in 2016, the California Energy Commission (CEC) met to began working on a new microgrid roadmap, created to encourage microgrid development in California. The roadmap identifies the top barriers to microgrid commercialization and examines ways to improve commercialization and standardization.
Initially, the CEC cited as barriers lack of policies and regulations that enable microgrids, plus interconnection rules that impose limitations on microgrids. The CEC has since awarded almost $80 million in grants.
Clustering microgrids
Another big moment in the history of microgrids came when Illinois regulators approved Commonwealth Edison’s microgrid cluster in Chicago in 2018. The $25 million project — the first utility-scale microgrid cluster in the nation — is designed to help teach utilities how to integrate microgrids with renewable energy resources and how to maximize the efficiency and value of two microgrids that interact with one another. The microgrid will directly serve more than 1,000 residential, commercial, and small industrial customers in the South Side of Chicago.
A national security play
Along with these programs that gave microgrids a boost, the US Military has been an enthusiastic early adapter of microgrids in efforts to ensure the power stays on in mission-critical operations. The military has for many years relied on small, isolated, self-contained grids in remote locations. More recently, the modern microgrid has altered the way the military and the federal government approach reliability and sustainability.
The federal government realized that a military base could install solar panels for some portion of its load to help achieve renewable goals while also making the base more resilient and self-sufficient. The Navy was one of the first branches of the military to build microgrids, installing one at the hospital Navy Base in San Diego. Since then, the military has installed several others including sophisticated projects at Marine Corps Air Station (MCAS) in San Diego and the US Marine Corps Recruit Depot (MCRD) Parris Island, South Carolina.
Evolution of microgrid financing
While microgrid awareness and interest was building among the military and others, acquiring the financing to build the microgrids was challenging. Commercial building structures, ownership and leasing arrangements all varied considerably, which made financing specific to the project and therefore difficult.
While it made sense logically to build microgrids, it didn’t yet make sense financially or operationally for many businesses. Financiers were not set up to finance projects that were small and specific to each individual project. This has changed in recent years because of models that derisk the investment for customers. These are offered under such names as microgrid-as-a-service, reliability-as-a-service, and energy as a service (EaaS).
These approaches convert a long-term capital expenditure to a short-term operational expense, thus keeping a large capital expense off a company’s books. Commercial customers can take on projects with no uprfont capital spending. Instead, a third party or financier typically owns the equipment and the customer pays a service fee, much like a monthly utility bill. Agreements vary on a case-by-case basis.
Product differentiation emerges
As microgrid use has expanded, so has its applications. Microgrids at first were viewed as a way to increase reliability, keeping the power on when the central grid failed. Their applications have widened into carbon efficiency. Wider adoption of microgrid technology has also been buoyed by cities, states, corporations and campuses that have set sustainability or carbon-emissions reduction goals. These have helped drive development of clean energy microgrids – those that incorporate renewables. Newer microgrids often use solar panels or wind turbines, and more are beginning to emerge that incorporate electric vehicle charging stations.
The military microgrid on Parris Island. Photo courtesy of Ameresco
Microgrids also are used to keep energy costs in check, as developers become increasingly adept at employing financing innovations and state and federal renewable energy incentives to lower energy costs. Sophisticated microgrids can participate in certain wholesale markets and leverage their assets to reduce costs.
As microgrid applications have expanded, they have entered the phase of product differentiation, which has led to many different types of microgrids, from fractal microgrids to virtual, blockchain, flying, sailing and more. At this time, NRG offers asset-backed demand response microgrids that focus on providing demand response and S&C Electric provides non-wires alternatives, which allow utilities to avoid investing in traditional poles and wires. And while microgrids continue to be highly customized products, the industry also is working on refining simple plug-and-play microgrids that can be manufactured in a replicable fashion and in some cases be installed in a day.
Today microgrids can be found at a broad range of commercial, institutional, industrial, community and government facilities. But residential microgrids remain rare, although some do exist, including one at the ranch of former California Gov. Jerry Brown. And some home developers are beginning to install neighborhood microgrids, but they too remain unusual.
Even though the history of microgrids spans for more than a hundred years, it’s been the last six that have brought growth in the leaps and bounds. Numerous drivers suggest it’s just the start of a lengthy buildup of microgrids in the US.
If you found this article on the history of microgrids helpful, subscribe to the free Microgrid Knowledge newsletter for more news and information about the growing industry.
Microgrid At The Center of $630 Million London City Airport Expansion
The London City Airport microgrid, now under development, will nearly double the size of the airport’s electricity distribution infrastructure, from 3.6 MVA to 7 MVA, and help power a major airport expansion and upgrade.
July 8, 2019 By Andrew Burger
The London City Airport microgrid, now under development, will nearly double the size of the airport’s electricity distribution infrastructure, from 3.6 MVA to 7 MVA, and help power a major airport expansion and upgrade.
Photo by Londons Finest Eye/Shutterstock.com
The microgrid is a critical element of the airport’s broader $630.5 million City Airport Development Program, which includes construction of a new terminal four times the size of the airport’s current terminal and the first digital air traffic control tower in the world for an airport of its size, The microgrid is scheduled to come online in phases through 2022 and into 2023.
The airport’s development transformation program “is central to the airport’s plans to help tackle the climate challenge and operate sustainably to achieve their net-zero carbon emissions goal by 2050,” said Tony Blackwell, design & interface manager for UK Power Networks Services (UKPNS), which operates the London City Airport’s existing electricity network.
Blackwell declined to provide the microgrid project’s total cost. He did explain that the cost of the microgrid will be incorporated within UKPNS’ existing Marketspur Agreement with London City Airport, which runs until 2033. UKPNS is designing, building, operating, maintaining and financing the microgrid.
One of most complex and demanding networks
UKPNS’ distribution management system will be the centerpiece of the microgrid and the airport’s new, much larger electricity distribution network, providing full remote control and systems automation and rapid fault response to the high-voltage distribution network.
“UK Power Networks’ ability to provide disaster recovery service from their control center will ensure that the operations are secure,” Blackwell said in an interview with Microgrid Knowledge. “The disaster recovery service is provided by an experienced team which controls the whole of the south east of the UK’s distribution network including London and will provide 24/7, 365 support to the dedicated UK Power Networks Services’ operations and maintenance team.
He added: “This experience is unmatched within our industry, since this network is one of the most complex, demanding and includes one of the most critical cities in the world with a very high availability rate of electrical infrastructure.”
The London City Airport microgrid will incorporate solar and combined heat and power (CHP) and a new, smart SCADA (supervisory control and data acquisition) system. It’s designed to enhance energy security and resilience while at the same time reducing infrastructure costs. The project also is designed to help improve the air quality around the airport and meet London’s citywide decarbonization goals.
The CHP and PV systems will be linked to the airport’s building management system, Blackwell said. Both systems are in their detailed design stages. Plans call for a solar PV system with a capacity of about 140 MWh and a CHP plant of 230 kW, as well as a second CHP unit of the same size in the future.
Microgrids are “Holy Grail”
Sustainable development is an airport priority. The facility has reduced its per-passenger carbon emissions 28% since 2013 and holds Level 3 Airport Carbon Accreditation. Its goal is to be carbon-neutral in terms of emissions by 2020. It is the closest airport to London’s city center and nearly 70% of the airport’s passengers arrive and leave via public transports — the highest proportion among all UK airports and something the airport actively encourages, according to UKPNS.
Airports in the US also have been showing interest in investing and deploying microgrids in recent years, a trend that picked up momentum in the wake of a 90-minute utility grid outage at Reagan International Airport in Washington, D.C. in August 2018 and an 11-hour power outage at Hartsfield-Jackson Atlanta International Airport in February 2018.
For example, AlphaStruxure, a joint venture of Schneider Electric and The Carlyle Group, is developing multiple microgrids as part of the modernization of JFK Airport in New York.
Free Resource from
Microgrid Knowledge White Paper Library
Microgrid Benefits and Example Projects
Microgrid benefits include energy reliability, energy accessibility, energy independence, and energy cost optimization. Download the new white paper from Schneider Electric that explores the benefits microgrids can provide and supplies examples of relevant microgrid projects.
In Pennsylvania, Allegheny County Airport Authority is planning a microgrid for the Pittsburgh International Airport. And in Tennessee, the Chattanooga Electric Power Board and partners are working on a “dynamic boundaries” microgrid with an open-source controller for the Chattanooga Airport that would link into existing automated switch gear and hence to the utility’s smart grid network.
“Microgrids are the holy grail of new sustainable renewable energy networks. Finding ways to make microgrids economically viable and self-funding has challenged global energy markets for decades,” said UKPNS Director Ian Smyth. “We are excited to deliver this turnkey solution for our valued client. Our ability to bring these technologies together delivers triple bottom line benefits for the airport — lower cost, greater resilience and help towards the UK’s decarbonization agenda.”
Track news about the London City Airport microgrid. Subscribe the free Microgrid Knowledge newsletter.
Detecting and Understanding Stray Voltage
Spark Your Electrical Vocabulary
All stray voltage is unintentional and undesirable, yet it is extremely common. In fact, it would be rare to find a farm or home without it, usually not in a good location. The main culprit, even though there are several variations of causation, is that with all standard 120 volt wiring we only have one hot wire, one neutral wire and a ground wire.
If the neutral wire is inadequate or if there is a weak or failed connection, the electrical current arriving on the hot wire must return to the source in some manner, which means it will try to go through any and all other objects that will conduct electricity. This undesirable flow of electrons can be via the earth, metal buildings, metal stanchions, fences or other objects.
The motor on a center pivot irrigation tower had been experiencing a tiny short in the wiring recently on a Midwestern farm. It had been this way for several weeks, but it was still working, and as you know there’s never enough time to do everything on the farm. However, the sand filter on the irrigator was also full, and this function needed emptying. The farmer was up on a metal ladder opening the overflowing trap to clean it out. It was safe, because all the pumps were switched off — except for what he did next, which was to instruct his wife to turn on the pump in order to flush the sand. It was a fatal mistake, as 480 volts surged through the system, instantly killing the farmer.
Another farmer had a grinder in the shop with a minor short in the motor; when it was turned on, it would give out a little shock. He “cured” the problem by turning on the grinder switch with a wooden broomstick. Who hasn’t done something like that?
On another farm there was a series of five livestock water fountains all connected to the electrical line. The first four fountains seemed normal, and the cattle were approaching them casually and drinking water normally. However, the cattle seemed to sense something was wrong with the fifth fountain, and they avoided it. Thirsty, two young heifers approached the fifth fountain, which was also overflowing slightly and creating a small puddle they were standing in. Within seconds after touching the water in the fountain, both heifers were instantly killed.
I heard many stories like this from Jerry Lush, a professional stray voltage consultant and ag engineer from Sioux Falls. After decades in the field of electrical energy, Lush can recount many horror stories of the abundant, and usually safe, power supply that we can’t seem to live without. Even folks who do not allow commercial electricity on their farm can encounter problems. I’m talking about stray voltage, a potential evader that can sneak onto any farm or barn.
What is Stray Voltage?
This is a very aptly named problem, in that it applies to any two objects that have electrical potential between them that ideally should not have any voltage difference between them. How much does it take? In general, we are always hoping for zero voltage, however, almost any animal can easily feel anything at 0.5 volts or higher. We could feel it too, but we usually have shoes or boots on and sometimes gloves. Lush says he finds this all too often and has even seen it run as high as 9 volts of current. Just imagine touching your tongue to a 9 volt battery.
Spark Your Electrical Vocabulary
Amperage: A measurement of the amount (strength) of current that is flowing through a wire.
Current: As stated above, current (flow of electricity) is measured in amps.
Induced voltage: A form of stray voltage that comes from other nearby circuits. This is more difficult to diagnose, but commonly runs through head stanchions or milk lines. It can be diagnosed and cured by a professional.
Resistance: This is something like a heater or light bulb; it is anything that holds back the current. It is measured in ohms.
Single-phase wiring: Brings 120-240 volts via one to two hot wires.
Three-phase wiring: (High voltage for large motors) brings in three hot lines.
Voltage: A unit of measurement of the pressure that pushes the amps through the wire.
Wattage: The sum of volts X amps and equal to power, as in the horsepower of an electric motor, for example. High voltage lines can adjust current flow by vastly increasing the voltage, which simultaneously lowers the flow of current and reduces line loss. Many transmission lines carry 7200 volts (this is what linemen work with) whereas coast-to-coast lines can carry 35,000 volts or more.
Potentially dangerous stray voltage was just diagnosed in our own home because the neutral wire coming from some “professionally installed” wiring, which had been put into our house by licensed electricians during remodeling, had actually been spliced into the ancient knob-and-tube neutral wire that runs through most of the walls and ceilings.
Jerry Lush has nearly 40 years of experience in the field of electrical energy.
Lush states that a big part of the problem is that electricians and linemen may see electricity in a different way than engineers trained in electricity (I’m generalizing here; there are some very knowledgeable technicians, likewise engineers are frequently so specialized they just don’t know everything, some engineers have no electrical training at all). But typically, the linemen have not been trained in household or farm wiring. Sometimes they can barely visualize the flow at all; their job is to get the power to the site.
Electrical engineers, including agricultural engineers, are trained to see electrical current wherever it is, quite like the rest of us might see water flowing. We could hardly expect to see water flowing into a structure without knowing where and how this water will exit. With voltage, if the neutral wire is not fat enough, or if the distance is too far, there’s no way it can keep up with electrical flow so that current “spills” into other areas in order for it to eventually get back to the source.
Stray voltage can come from any electrical device that is malfunctioning. Even properly installed wiring or devices can be damaged by moisture, lightning, or mice, squirrels and rats. Most commonly afflicted are barn fans in the summer and water tank heaters in the winter. Lastly, there can often be problems coming onto your farm from the utility service. Wherever the source, proper diagnosis is a critical starting point.
Symptoms of Stray Voltage
The key word is mysterious. Many farmers think they must be bad farmers or bad managers, or that they must have poor-quality livestock, not realizing there is a hidden cause. Electricity is essentially invisible, and we are usually focused on visible issues. Every single farm, ranch barn, garage or home can have stray voltage problems — we have seen it with dairy, beef, swine, sheep, goats, poultry or horses, but most often electrical problems are most clear in a dairy. In general, dairy animals drink more (to make milk), and they are quite often indoors and being handled, in a place where we can watch them.
Animals that are plagued with stray voltage will most frequently manifest specific problems such as mastitis, or high somatic cell count (pus in the milk), or they are jumpy when they come in to be milked. In many cases they just will not let down their milk flow. Watch your animals when they drink; they will tell you. Frequently they will only drink just enough to satisfy their thirst but not enough to maintain adequate production, which soon falls off even worse. Instead of taking a steady intake of water, they merely lap at the water, bobbing their heads.
Humans are more likely to feel the voltage themselves when walking barefoot on wet concrete, even more so when touching plumbing or metal when they are somewhat grounded by being wet. People have even been known to keep a dry rag around so that they can shut off their shower faucet without getting a mild shock.
Diagnosing Stray Voltage
Ideally, hire a pro! Lush is one of several in the United States. He comes by his skills honestly with two degrees in ag engineering and years of service working for rural electric utilities and co-op extension services. He has focused exclusively on stray voltage problems since 2007. Having worked both for the utility and for the farmer, he understands both sources of problems. He says his main tool of the trade is a simple volt meter, one that can measure micro voltage. At times he will hold a metal rod in one hand as he explores with the leads from a volt meter. He also uses a device that converts electrical current into an audible signal which emits a buzz if there is current flow. Quite often he can instantly spot wiring design errors or find loose connections. By the use of all these devices he can pinpoint sources of the problem.
Electric fencing is rarely a problem, in general, but if wired wrong it can be devastating. Lush says that it is of utmost importance to create a grounding system that is as good as or better than that of the rest of the farm. The fence should have its own individual ground and it should never be attached to any other ground. Place the ground far away from barns or other electrical systems.
Can Stray Voltage Be Cured?
Absolutely! However, Lush admits there are a few mysterious challenges over a lifetime of work. He recalls a few farms that defy logic such as an Amish farm he once investigated that haunts him. They were having barn issues of serious stray voltage in the metal stanchions yet were hundreds of yards from power lines, buried lines, transformers or substations. In some of these cases, even though no source can be detected, the professionals can build a circular passageway around the farm buildings using highly conductive materials.
Most of the time however, he says he can diagnose and cure almost every farm within four hours’ time, and most diagnoses come in the first half hour. Even if the problem is coming from the utility, a power pole/transformer neutral isolator can be installed. Since many problems come from inadequate grounding, this is a cure that can be rewired in a proper manner and without much cost. With 240 volt wiring there are fewer problems because there are two hot wires, and the current will arrive via one hot line and go back to the source via the other hot wire.
However, it’s not always that easy to settle disputes if questions arise with regard to the sources of the problem. If the utility will not accept responsibility for causing the problem or for the cost of fixing it, many farmers can feel left in the lurch. In fact, many institutions practically deny the existence of the problem, some even insinuating that the farmer must either be crazy or just a whiner.
Here in my state of Minnesota alone there are currently at least six pending lawsuits between farmers and the utilities with little hope of resolution in sight. However, the tide is slowly beginning to shift toward more accountability and more willingness to admit that the problem exists. Is it worth fighting? One dairy farmer in Minnesota suing the power utility estimates the voltage running through his dairy cost him over $700,000 in lost production, last year alone. Another Minnesota suit was settled, awarding $3 million to the damaged parties.
Second Greenhouse Heated by Cryptocurrency Mining
UnitedCorp's technology uses the heat from cryptocurrency mining to support greenhouse agricultural operations
Miami-based United American Corp announces the completion of its second BlockchainDome and the full commissioning of 1,500 additional miners for a total of 2,500 miners (3.8 megawatts) now in service in two BlockchainDomes. Pre-installation of 1.5 megawatts of electrical service for adjacent greenhouses heated by the BlockchainDomes is now also complete.
The latest BlockchainDome incorporates a number of improvements in construction and deployment from the first dome which includes mass pre-fabrication of a number of dome components and in-house CNC manufacturing of the mining rig docking stations. Construction logistics have also been refined to include pre-installation of foundations and utilities for future domes resulting in overall lower construction costs and shorter construction timelines.
"We have taken everything we have learned from the construction of the first BlockchainDome and used this knowledge to make the implementation of this subsequent BlockchainDomes faster, cheaper and of better quality," stated UnitedCorp CEO Benoit Laliberte. "Along with the generation of heat from the BlockchainDomes for agricultural purposes, our goal remains to be the low cost and environmentally sustainable standard for the industry."
UnitedCorp's technology uses the heat from cryptocurrency mining to support greenhouse agricultural operations through the BlockchainDome Heat Station system which keeps greenhouses at 20oC year-round. This represents a simple design solution compared to various alternatives whereby the cost of generating this heat from a single source is shared between multiple use cases.
Commercial greenhouses in cooler climates like in the Province of Quebec typically require a significant amount of thermal energy to supplement daytime solar energy, particularly during the period of September to May, and many older greenhouses utilize inefficient heating systems for this purpose. The dry heat produced by the BlockchainDome Heat Station is also used in the summer to reduce greenhouse mold and fungus caused by condensation thereby reducing or eliminating the need for chemicals to treat this problem and creating a more organic growth environment.
UnitedCorp believes this "Heat Campus" approach for heat generation and utilization is the future for agriculture and any other industry that can make use of low-cost heat with the ultimate goal being to get as close zero waste as possible. This is not only good economically but allows businesses to "green" their operations by significantly reducing the amount of electricity the combined operations require from the grid.
For more information:
UnitedCorp
5201 Blue Lagoon Drive, 8th floor,
Miami FL 33126
www.unitedcorp.com